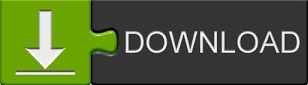
(17) It can be also stored as a liquid with a higher volumetric energy density at much lower temperatures (−253 ☌ at 1 bar). (16) Nowadays, hydrogen is commonly stored as compressed gas at pressures up to 700 bar at 25 ☌. Furthermore, hydrogen tends to diffuse through the materials, leading to embrittlement or weakening of the storage material. Hydrogen has a very high energy density by mass (119.7 MJ kg –1 of lower heating value at 25 ☌ and 1 bar (14)), but it has a very low energy density by volume (8.96 GJ m –3, referred to as liquid fuel (15)). (13)Īnother of the current challenges in hydrogen technologies is its storage and transport. PEMFCs are the most widely used for mobility and small applications. The most common fuel cells are solid oxide fuel cells (SOFC), proton exchange membrane (PEMFC), direct methanol (DMFC), alkaline (AFC), phosphoric acid (PAFC), and molten carbonate (MCFC) fuel cells. Currently, there are several commercialized fuel cell-based technologies that differ mainly in the electrolyte, fuel and/or working temperature.
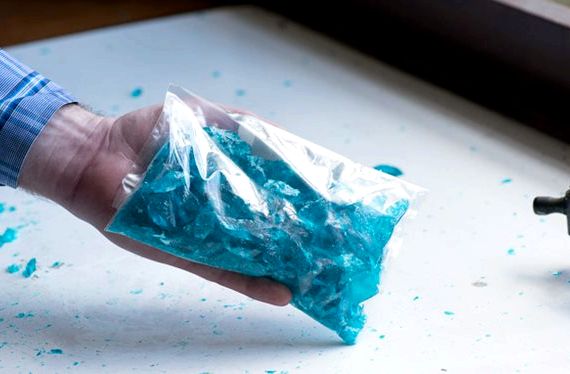
Moreover, fuel cells have the ability to continuously produce electricity while fueling them, which is a significant advantage compared to batteries. (12) Hydrogen represents an effective and clean alternative to the use of fossils fuels, which have a high carbon footprint, to produce energy by using fuel cells, since water is the only product generated in the process, apart from heat. (11) Currently, most of the hydrogen is produced and used on-site in industry, principally for ammonia production and petroleum refining, which together account for two-thirds of the total hydrogen use. (10) Nevertheless, a large reduction in the cost of electricity from renewable sources and electrolyzers is needed to allow the hydrogen produced by electrolysis to compete with conventional sources of energy on a large scale. Although the electrolysis of water is a well-known and established technology to produce clean and high purity hydrogen, (9) it comprises high energy losses. Nowadays almost all the hydrogen produced comes from catalytic steam reforming of fossil fuels, mainly from natural gas, (7,8) which is currently a well-established commercial technology and is the least expensive way to produce hydrogen on a large scale. Finally, the structured reactors used to date for the decomposition reaction of ammonia are explored, as well as the possibilities offered by catalytic membrane reactors, which allow the on-site simultaneous production and separation of hydrogen.
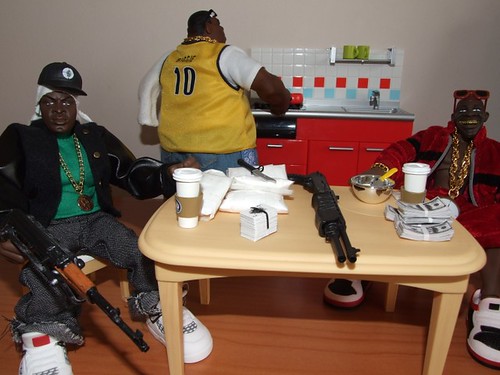
An analysis of the kinetics of the reaction obtained with different catalysts is also presented and discussed, including the reaction mechanism, the determining step of the reaction, and the apparent activation energy.
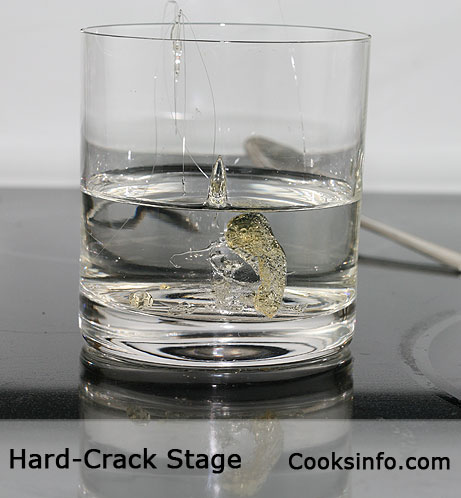
The effect of the supports and promoters used is analyzed in detail, and the catalytic activity obtained is compared. Then, the state of the art of the catalysts used to date for ammonia decomposition is described considering the catalysts composed of noble and non-noble metals and their combinations, as well as novel materials such as alkali metal amides and imides. First, an analysis of the existing systems for ammonia decomposition and the challenges for this technology are presented.
#How to cook up crack with ammonia portable#
Because of the problems associated with the generation and storage of hydrogen in portable applications, the use of ammonia has been proposed for on-site production of hydrogen through ammonia decomposition.
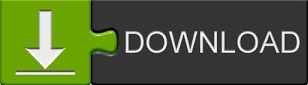